In many engineering fields of data processing, such as deep space object detection and tracking, people need to detect features, such as edge and shape information of the asteroid targets and celestial surfaces, from incomplete measurements limited by conditions of physical constraints or high cost of the instrument.
Dr. SHAN Hao from the Xinjiang Astronomical Observatory of the Chinese Academy of Sciences has conducted theoretical research on multi-object edge detection in highly incomplete measurements using the curve evolution theory of differential geometry, and based on fast optimization algorithms of compressed sensing.
The study was published in Pattern Recognition Oct. 5.
In this study, the researchers proposed a new method and its algorithm is actually a three-step process, including edge detection, active contouring and sparse reconstruction. The detection is not carried out completely after optimization on the reconstructed data, but rather recovers edge information from intermediate measurements during the optimization.
From the evaluation indicators (peak signal-to-noise and root mean square error) of the numerical experiments, the proposed method has advantages over some of the state-of-the-art methods. These advantages include: the useful information of intermediate measurements is fully exploited to improve the accuracy of the detector; the detector derived from the complex geometric wavelet transform achieves an optimal edge approximation rate.
“In future studies, based on the current mathematical framework, we will apply our research results to the decoding stage of deep space remote sensing, and the radio source detection in high dynamic range and large field-of-view radio scenes,” said Dr. SHAN.
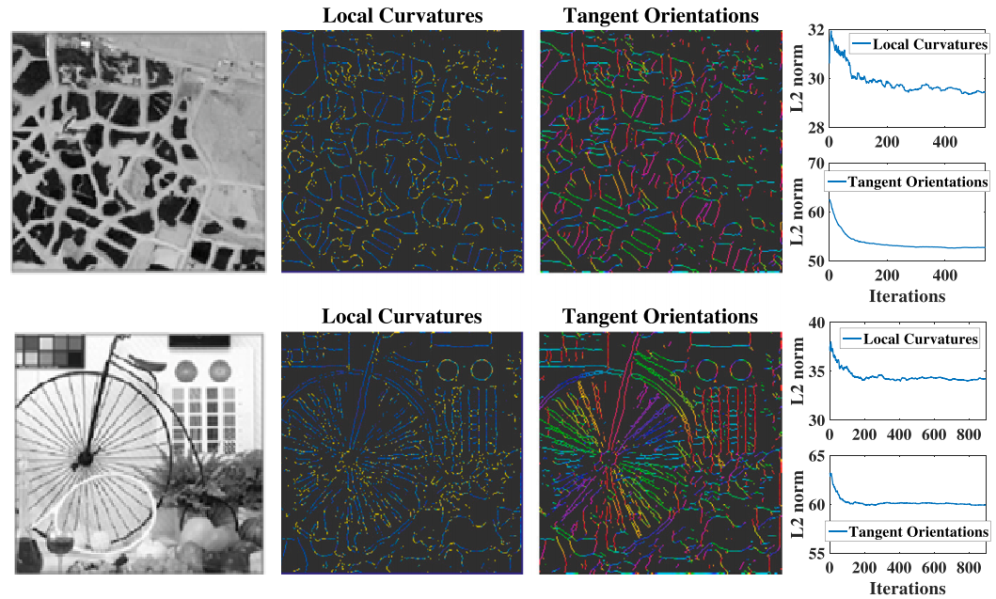
Fig. 1. The local curvatures and tangent orientations. Column 2: Darker line segments denote curvatures closer to zero degrees. Column 3: Red and green line segments indicate vertical and horizontal orientations, respectively. Column 4: convergence processes of the two geometric features. The reconstruction is from spectral measurements taken on 33 radial lines.
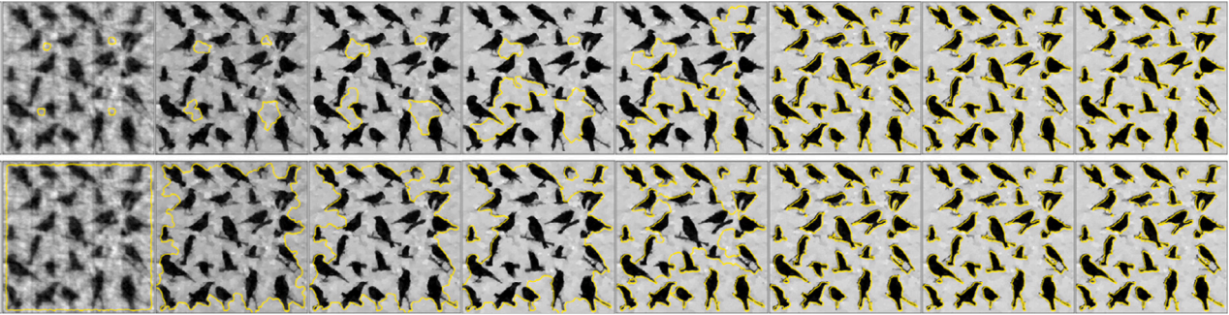
Fig.2. The CS-GAC evolution in case of a large number of objects. Row 1: outward evolution with four initial contours. Row 2: inward evolution with one initial contour. The spectral measurements are taken on 17 radial lines (sampling rate:0.0728). The images from left to right are selected from iterations 2, 100, 250, 400, 500, 800, 1100, and 1129, respectively.
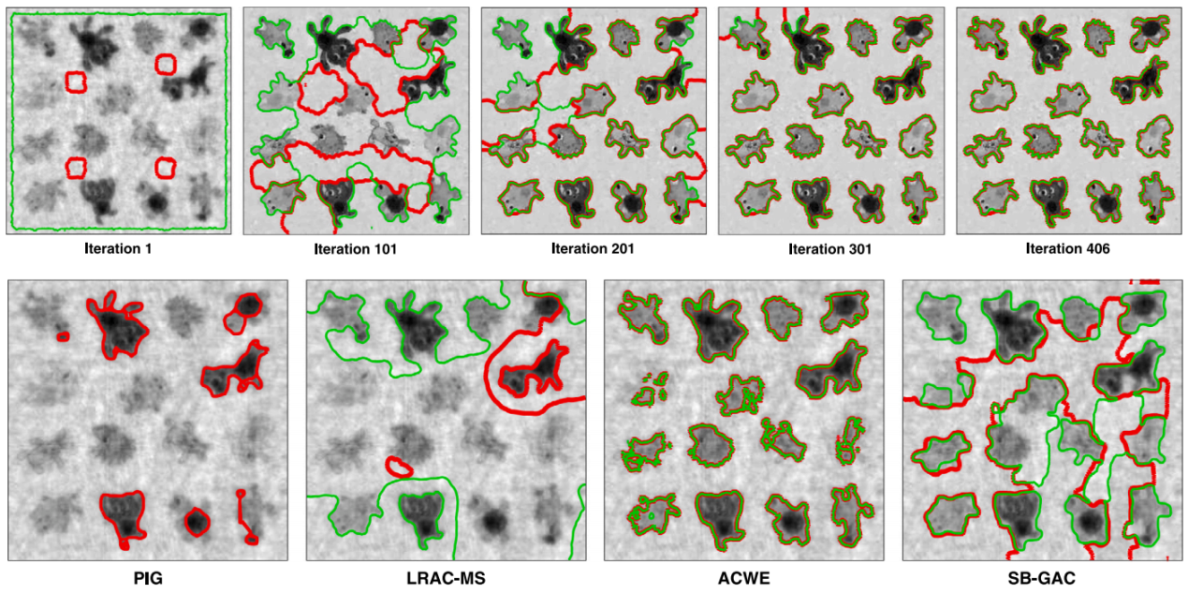
Fig.3. Segmentation comparisons of weak objects between the CS-GAC and four state of the art methods. Reconstructions are from spectral measurements taken on 27 radial lines (sampling rate:0.1145).
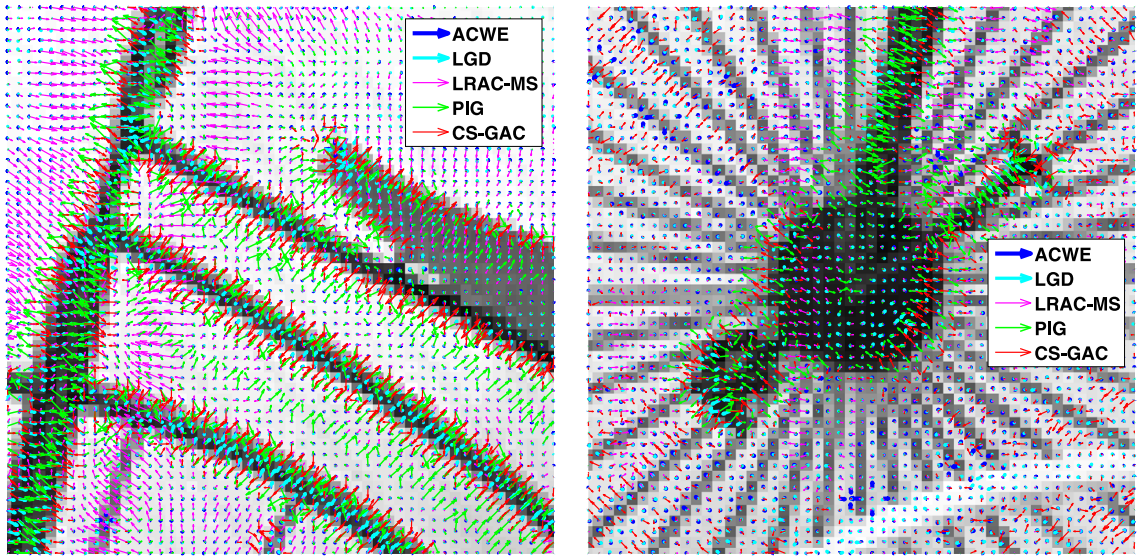
Fig.4. Comparisons of the external potential force fields among the CS-GAC and four state of the art methods. The reconstruction is from spectral measurements taken on 77 radial lines (sampling rate:0.3074).
Contact: SHAN Hao
Xinjiang Astronomical Observatory, Chinese Academy of Sciences
Email: shanhao@xao.ac.cn
Article link: https://www.sciencedirect.com/science/article/pii/S0031320323007045